by K. Weber and C. Mattheck
The consequences of excessive drilling on the trunk using an increment borer and a drilling resistance measurement device were determined for various tree species. The examined trees had internal decay (soft rot, white rot, or decay cavity), which were also indicated externally by defect symptoms such as bulges, open wounds with callus formation. In comparison, a healthy tree, i.e., free from decay, that had been extensively drilled in the past was also investigated.
The selected trees were all “experimental trees” from previous field studies, which had been drilled multiple times, or annually, using an increment borer and IML-RESI (drilling resistance measurement device) eight to ten years ago.
The following questions were to be answered:
- How does a tree with internal decay react to the multiple or extensive use of invasive diagnostic techniques?
- Do decays from the inside (decay cavity, internal decay) emerge through the drill holes, or does decay from the outside enter the trunk, or both?
- How does a healthy tree react to the multiple or excessive use of invasive diagnostic techniques?
- Are drilling techniques “dangerous” for the trees?
- Are there differences in the potential harmfulness of different drilling techniques?
- Does the tree inspector need to drill at all?
Drill sizes:
The cutting diameter of an RESI drilling needle was 3.0 mm. The outer diameter of the increment borer, which produced a 5 mm core sample, was 11.3 mm.
Inhalt
Basics
Drilling Techniques[1]
For a thorough examination of fungus-infected trees, various drilling techniques can be applied depending on individual circumstances. The use of an increment borer represents the method with the highest informative value in this regard. The visual assessment of the core sample provides extensive information about growth rates and decay compartmentalization in the tree. Wood discoloration without actual wood decay, such as those occurring after shaft damage, can also be detected in most cases. Qualitative (wiggle test) and quantitative (fractometer) fracture tests of the core sample provide insights into fracture behavior (brittle fracture, tough fracture) and individual wood strengths. Furthermore, the core sample can even be assessed through smell (wet core!) or subjected to detailed microscopic and biochemical analysis in a wood laboratory. Drilling resistance measurement is arguably the fastest of all drilling techniques. During the relatively quick measurement process, a recordable drill graph is produced. This drilling technique is particularly suitable for detecting dangerous wood embrittlement caused by brown rot or advanced soft rot as a means of proof. Even finer quality differences in healthy wood, such as earlywood and latewood in conifers, can be well recognized.
Finally, the use of a spiral drill bit should be mentioned, which is a drilling technique that allows investigation of even the hardest tropical woods that cannot be penetrated by an increment borer or drilling needle. With the spiral drill bit, shavings are drawn at different drilling depths, which are long in soft and tough wood and short in hard and brittle wood. Wood discoloration can also be visually detected long before the wood begins to decay.
A general disadvantage of drilling techniques is the creation of a more or less large borehole. The tree is locally wounded and must heal this wound. (cf. Fig. 1)
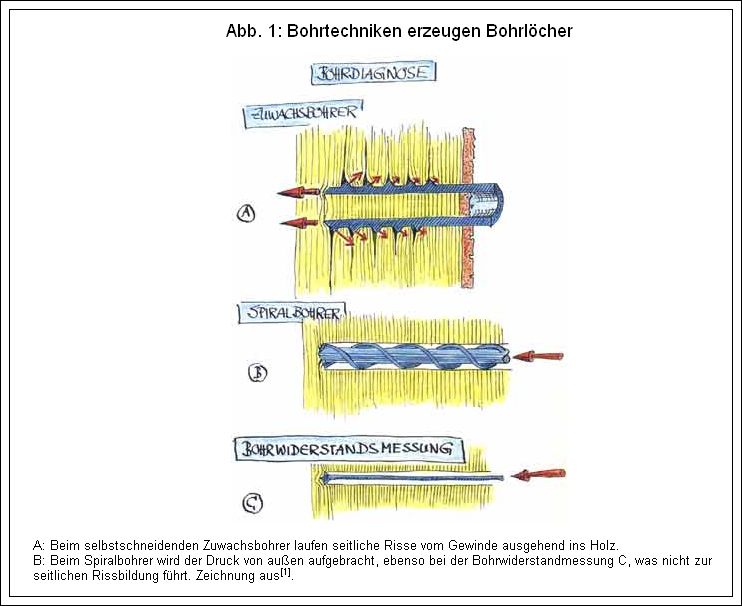
Fig. 1 Drilling techniques create bore holes
- With the self-cutting increment borer, lateral cracks run into the wood starting from the thread
- In the twist drill, the pressure is applied from the outside, as well as in the drilling resistance measurement C, which does not lead to lateral cracking.
Wood Decay and Compartmentalization[1]
In 1977[2] and 1979[3], Alex Shigo et al. described the mechanisms of decay compartmentalization in living trees in a model known as the Compartmentalization Of Decay In Trees (CODIT) theory. CODIT occurs when a fungus penetrates the sapwood from the outside after the cambium has been injured. The invading decay pathogen is impeded from spreading in the sapwood by a total of four walls (Wall I – IV).
Wall I
This wall hinders decay spread in the axial direction, both “above” and “below” the decayed area, by closing off the conducting elements and depositing substances that are inhibitory to fungi. Deciduous trees often form tyloses (see Fig. 2), and some also deposit gum-like substances as membranes or plugs in their vessels. Coniferous trees can seal their tracheids by closing their pit membranes. The existing upper and lower cell walls also act as axial barriers. The faster a tree builds Wall I, the shorter the columnar decay area in the wood, and thus the longitudinal extent of the decay.
[1] Note: The citation “Holzfäulen und deren Abschottung” refers to a section or source within a larger document or study. [2] Shigo, A. L. et al. (1977). CODIT: A Durable System for the Compartmentalization of Decay in Trees. Journal of Arboriculture, 3(11), 176-181. [3] Shigo, A. L. et al. (1979). Compartmentalization of Decay in Trees: A Decade of Research. Forest Pathology and Wood Microbiology Research, 49-56.
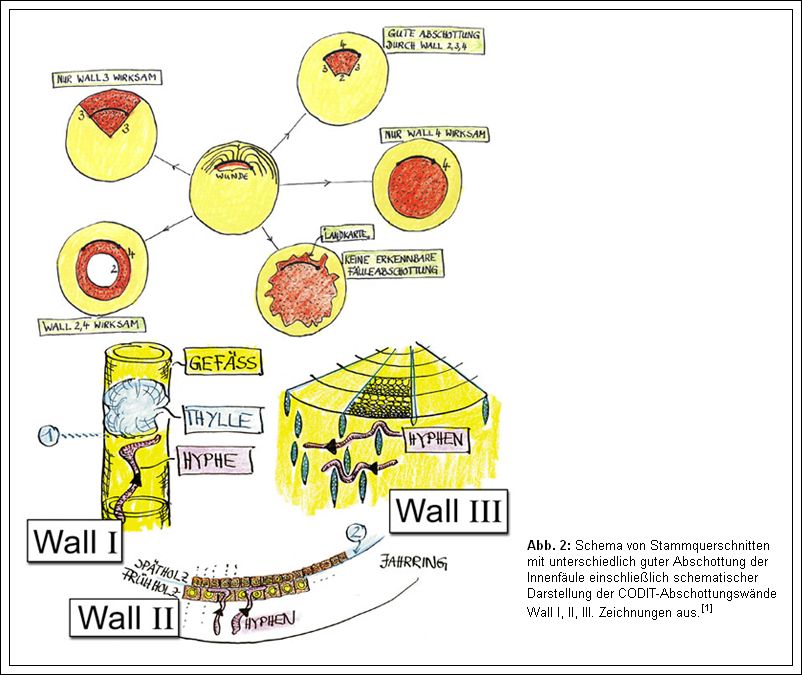
Fig. 2 Schematic of log cross-sections with different good compartmentalization of internal rot including schematic representation of CODIT compartmentalization walls Wall I, II, III.
Wall II
This wall hinders decay spread in the radial direction through the latewood of past years. At each annual ring boundary, the thin-walled earlywood cells abruptly meet the thick-walled latewood cells of the previous year, which are usually less extensively colonized by fungal hyphae (see Fig. 2). Additionally, living parenchyma cells are often present as tangential bands in the latewood or at the annual ring boundaries, which can further inhibit hyphal growth through the production of substances inhibitory to fungi. However, this is generally only possible in the sapwood since during the process of heartwood formation, all wood cells die.
Wall III
This wall hinders decay spread in the tangential direction, i.e., “to the right” and “to the left” of the decayed area, through the rays in the wood. The living ray cells in the sapwood produce defense substances, and their spindle-shaped structure also serves as a mechanical barrier. As the rays vary in height and are tangentially offset, Wall III does not form a continuous tangential barrier but rather consists of individual interrupted obstacles that the hyphae must encircle or break through, thus delaying the spread of decay in the direction of the annual rings (see Fig. 2).
Wall IV
Referred to as the “barrier zone,” it consists of a specialized parenchyma-rich tissue formed by the cambium after injury, which accumulates fungal defense substances in its cells. This zone is often much larger than the wound itself and frequently encompasses the entire circumference of the trunk (see also [5]). Through the highly effective barrier zone, the decay pathogen and the previously formed wood, in which the pathogen partially resides, are separated from the newly formed wood. The barrier zone often forms a sharp boundary between healthy wood and completely decayed wood.
Since the compartmentalization walls, Wall I – III, generally represent preexisting structures of the sapwood (excluding tyloses) that are chemically altered during the defense process, they are collectively referred to as the “reaction zone.”
In contrast, the barrier zone, Wall IV, represents both a chemical and anatomical defense reaction, as it contains many metabolically active cells (parenchyma cells) that produce and store fungistatic substances.
Fungi that enter a tree trunk without damaging the cambium, for example, through injured roots or at branch breaks or pruning wounds, likely do not induce the formation of a barrier zone (Wall IV) in the trunk. This is supported by the map-like decay contour (see Fig. 2), which is often found in root-infected decay. In such cases, only a reaction zone forms in the sapwood. The heartwood cannot actively respond to a fungal attack as it consists of dead wood. However, it is relatively resistant to many fungal species due to the process of heartwood formation, involving the deposition of polyphenols, resins, lignin, suberin, and other compounds in the cell walls.
Material and Method
The experimental trees:
- Black Poplar (Populus nigra L.) with extensive internal decay and cavity, defect symptom: swelling at the base of the trunk (with “elephant foot” – i.e., indentations between the root attachments were no longer visible), many RESI drillings, some increment borings. Decay pathogen: Honey fungus (Armillaria spp.).
- Sycamore (Acer pseudoplatanus L.) with crust fungus (Ustulina deusta) inside, very extensive internal decay, partially overgrown wound with barrier zone at the base of the trunk, many RESI drillings, and many increment borings.
- Oak (Quercus robur L.) with smaller internal decay and crack. Defect symptom: swelling on the trunk in front of the decayed area, rib formation in front of the crack, many RESI drillings, and increment borings.
- Sycamore (Acer pseudoplatanus L.). Leaning Norway maple with many increment borings and some RESI drillings on the tension and compression sides of the bend (through the trunk) at the base of the trunk or at the root collar. No internal decay prior to drilling.
The experimental trees were felled to subsequently cut out and examine tree disks from the relevant stem areas. The identified decay compartmentalization zones were verified under a light microscope. The materials and methods for microscopic examination were described in detail in[5].
Results and Discussion
First, the overall impact of excessive drilling, i.e., multiple drillings per year for more than 10 years, will be presented using a resistance drilling device (less frequently an increment borer) based on the fresh cross-section after felling the black poplar. Figure 3 shows the examined black poplar with a swelling at the base of the trunk, which externally indicated extensive internal decay and was also confirmed by the frequently applied drilling techniques. The excessively drilled area is highlighted in Figure 3.
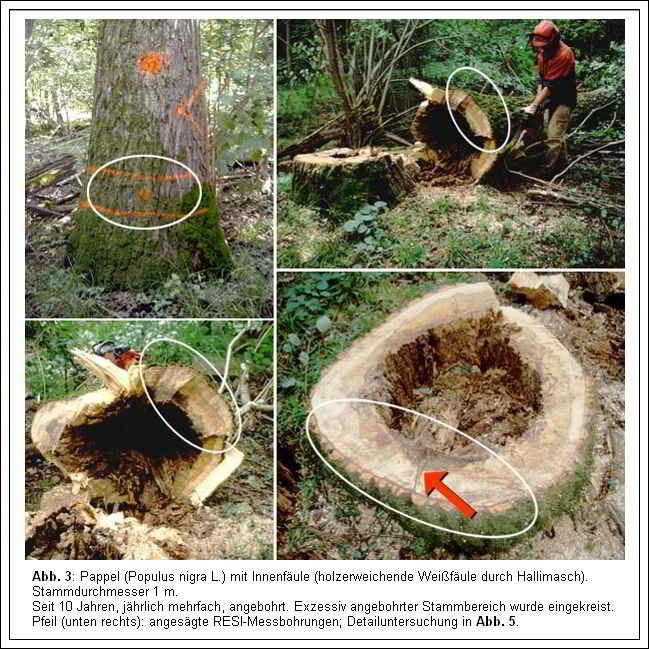
Fig. 3 Poplar (Populus nigra L.) with internal rot (wood-softening white rot caused by Hallimasch). Trunk diameter 1 m. Drilled for 10 years, several times a year. Excessively bored stem area was circled. Arrow (bottom right): sawed RESI measuring holes; detailed examination in FIG. 5.
The remaining wall thickness of the excessively drilled stem area (approximately 50-70 mm free from decay) did not differ significantly from the remaining wall thickness of the rest of the non-drilled stem area. This means that the numerous drillings, which locally penetrated the decay compartmentalization walls (e.g., reaction zones, possibly also barrier zones) from year to year, did not significantly affect the stability or resistance to breakage of the tree. Overall, the internal decay was not significantly pulled out of the excessively drilled area through the drill holes to destroy the outer, still healthy remaining wall (see also Figure 4).
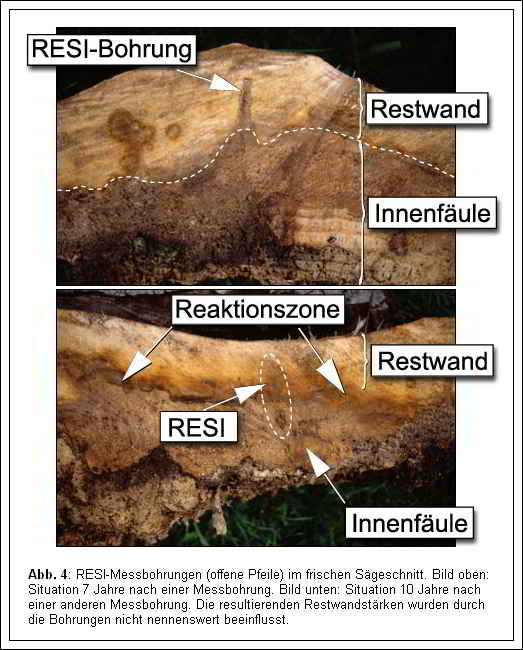
Fig. 4 RESI measuring holes (open arrows) in fresh saw cut. Picture above: Situations 7 years after a measuring hole. Picture below: Situation 10 years after another measuring hole. The resulting residual wall thicknesses were not significantly influenced by the drillings.
After evaluating the overall impact of years of drilling on the tree trunk, the changes in the wood structure caused by individual drilling will now be presented. Figure 5 shows the remaining wall in the area surrounding a 3 mm RESI measurement hole, six years after drilling. A relatively sharply defined reaction zone has formed in the poplar sapwood around the drill hole. The drilling hole was closed again by the subsequent annual ring formation within six months, with the cambium initially forming a thin, yellowish-brown layer, a “barrier zone,” around the drill hole opening. After six annual rings had formed over the drill hole without any external decay penetrating, it became apparent that internal decay had started at the inner end of the drill channel, locally decomposing the wood around the drill hole. Thus, it can be inferred that due to the drilling, the internal decay traveled slightly faster through the reaction zone formed by the tree than in the adjacent non-drilled area.
The entry and slow progression of decay into the drill channel remained relatively confined and triggered a strong formation of reaction zones in the sapwood. Simultaneously (within 6 years), the internal decay itself expanded within the poplar trunk, pushing forward a concentric reaction zone. An original reaction zone (RZ 1 in Figure 5) was gradually invaded by the decay pathogen, leading to the formation of a new reaction zone (RZ 2) in the radial sapwood area lying in front of it, and so on. The degree of decomposition of the “left-behind” decayed wood increased inward, ultimately resulting in the widening of the pre-existing central decay cavity.
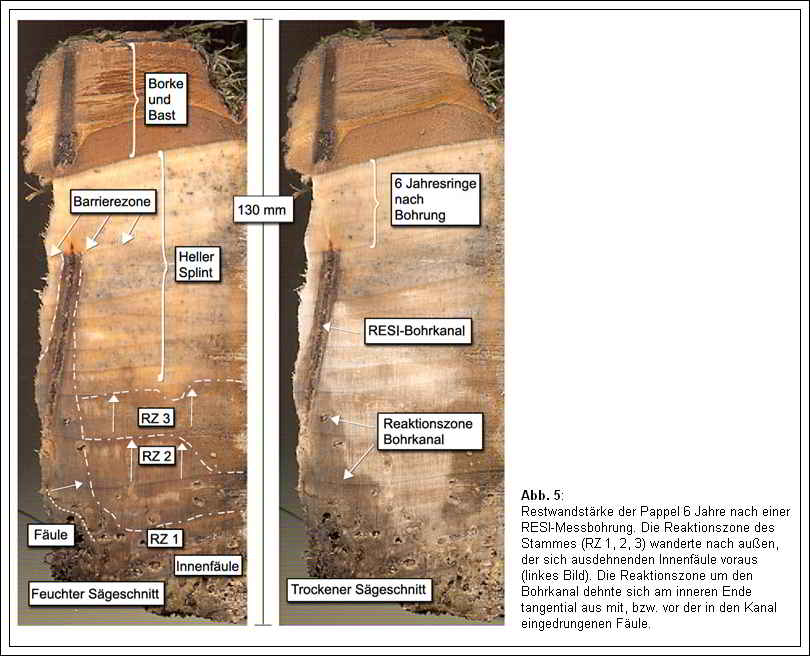
Fig. 5 Residual wall thickness of poplar 6 years after a RESI monitoring well. The reaction zone of the trunk moved outward, ahead of the expanding internal rot. The reaction zone around the bore channel expanded tangentially at the inner end with, or ahead of, the rot entering the channel.
After 6-7 years, as can be seen in Figures 4 and 5, the general, encompassing reaction zone around the central internal decay almost reached the radial extent of the decay pulled into the drill channel. After 10 years (Figure 4, lower image), the general, encompassing reaction zone surpassed the decay pulled into the drill channel, completely compensating for the local negative effect of the drilling.
Remarkably, the extent of reaction zone formation around the drill channel in the sapwood, caused by the drilling itself (i.e., mechanical destruction during drilling), as shown in Figure 5 (right image), was visibly smaller than the extent of reaction zone formation at the inner end of the drill channel, triggered by the locally invading decay. The tree seems to conserve energy here and only develops a thick “energy-intensive” reaction zone when it is necessary to prevent the actual decay pathogen from spreading.
This observation suggests that fungus-induced reaction zone formation is more pronounced and likely more effective overall than purely mechanically-induced reaction zone formation without fungal infection. In other words, where the reaction zone is truly needed in the tree, it is better than where it was only precautionarily established (or contributes to the prevention of air embolism).
The thicker the reaction zone, the more difficult it is for the decay pathogen to grow through it, meaning the inhibitory effect on decay spread is stronger. Since a reaction zone was formed along the entire drill channel in the poplar, which can be several centimeters long, as well as tangentially adjacent to the rear area of the channel due to the invading decay, it is locally (at the drill site) very thick in the radial direction, exerting a particularly inhibitory effect on radial decay spread. Therefore, it was likely possible for the concentrically expanding internal decay to catch up with the locally “pulled out” decay.
Since all the drillings found on the poplar exhibited the described patterns (barrier zone, reaction zone, etc.), a general and schematic representation of the “consequences after drilling” was subsequently developed (Figure 6, Figure 7, Figure 8, Figure 9).
In no case did decay penetrate into the drill channel from the outside and establish itself in the outer area of the drilling.
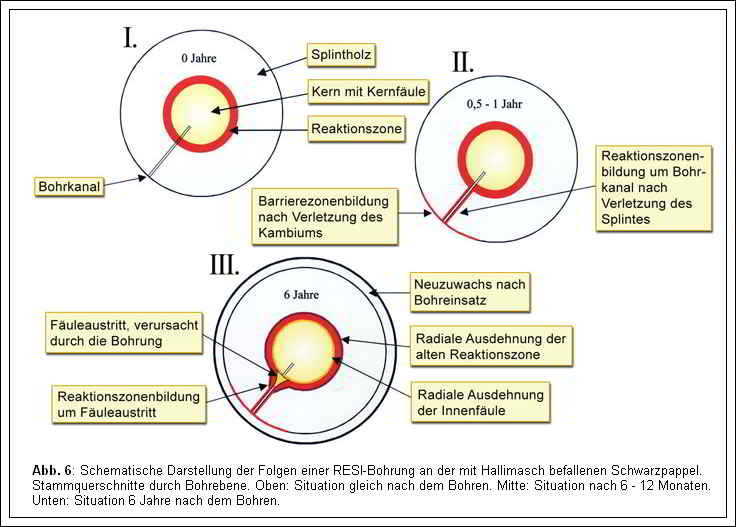
Fig. 6 Schematic representation of the consequences of RESI drilling on black poplar infested with Hall’s Mash. Cross-sections of trunks through drilling plane. Top: Condition immediately after drilling. Middle: Situation after 6-12 months. Bottom: Condition 6 years after drilling.
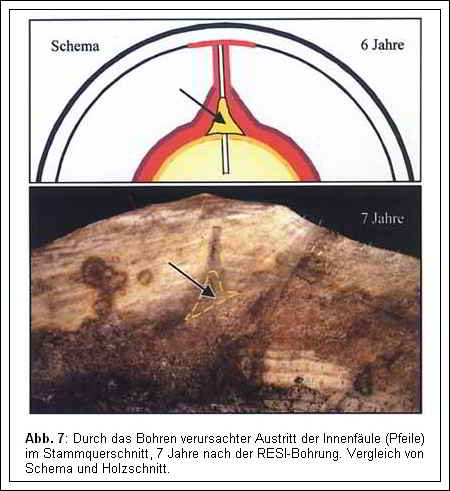
Fig. 7 Drilling-induced exit of internal rot (arrows) in stem cross-section, 7 years after RESI drilling. Comparison of schematic and wood section
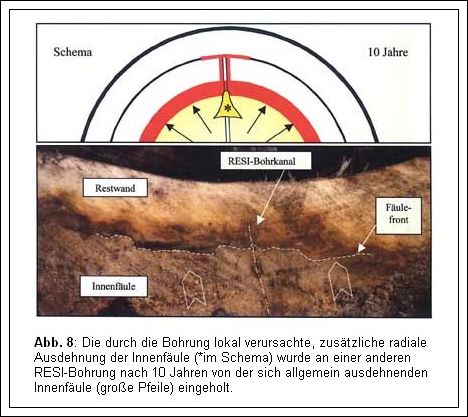
Fig. 8 The additional radial expansion of internal rot caused locally by the well (*in the schematic) was caught up by the generally expanding internal rot (large arrows) at another RESI well after 10 years.
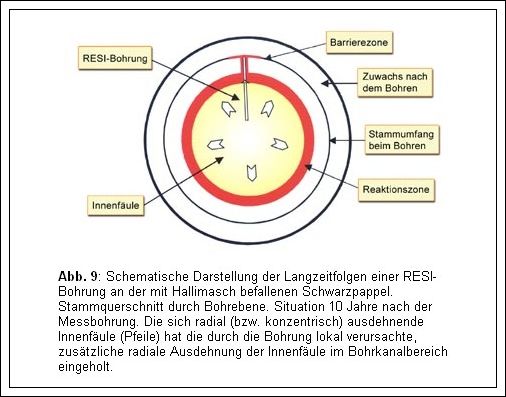
Fig. 9 Schematic representation of the long-term effects of RESI drilling on black poplar infested with Hall’s Mash. Cross-section of trunk through drilling plane. Situation 10 years after the measurement drilling. The radially (or concentrically) expanding internal rot (arrows) has caught up with the additional radial expansion of internal rot in the drill channel area caused locally by the drilling.
Next, we want to examine the effects of drilling along the axial direction, i.e., along the stem axis. Figure 10 shows a wood chip taken directly next to a RESI drill channel. The induced barrier zone (BZ) and the reaction zone above and below the drilling are clearly visible. In Figure 11, the changes in the wood structure of the poplar, six years after a RESI drilling operation, are depicted in cross-section and longitudinal section. Here too, the “walls” of the decay containment in the tree could be observed. The barrier zone and the reaction zone induced by the drilling, as well as the reaction zone formed by decay entering the drill channel, and the entry point of decay itself were much more extensive in the axial direction (parallel to the wood fibers) than in the radial direction (perpendicular to the wood fibers). This pattern was generally observed in all the identified drillings, which is why a schematic representation of the drilling effects in the axial direction was developed, as shown in Figure 12. Finally, the two schematic representations, Figure 6: Consequences after drilling in the stem cross-section, and Figure 12: Consequences after drilling in the longitudinal section, were combined into a three-dimensional schematic representation. Figure 13 A and 13 B illustrate the effects of drilling on wood decay in the tree, 6 and 10 years after a RESI drilling, as a 3D diagram.
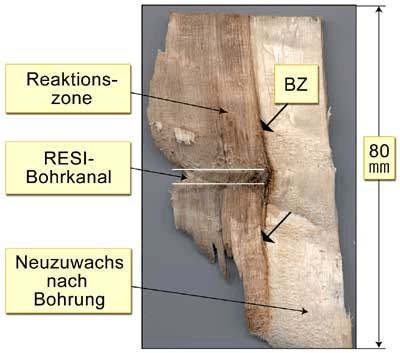
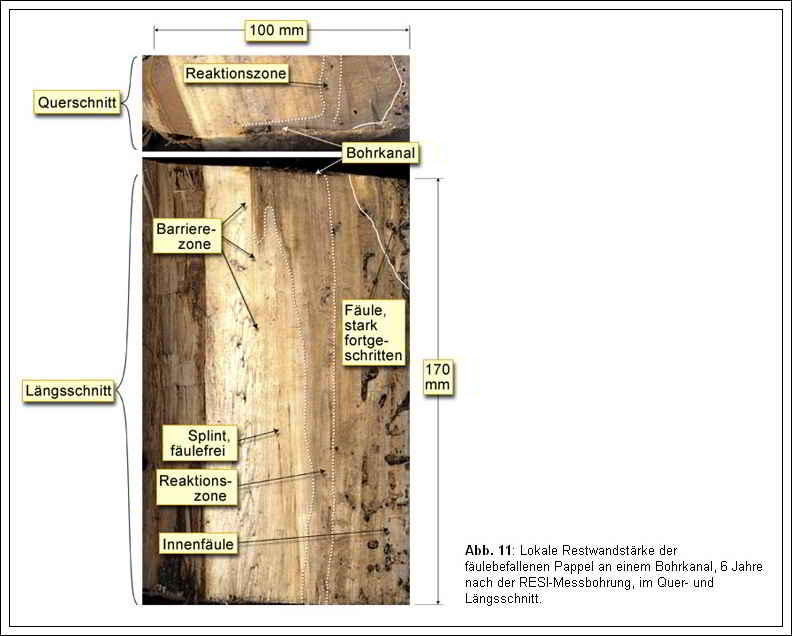
Fig. 11 Local residual wall thickness of decay-affected poplar at a drilled channel, 6 years after RESI measured borehole, in transverse and longitudinal sections.
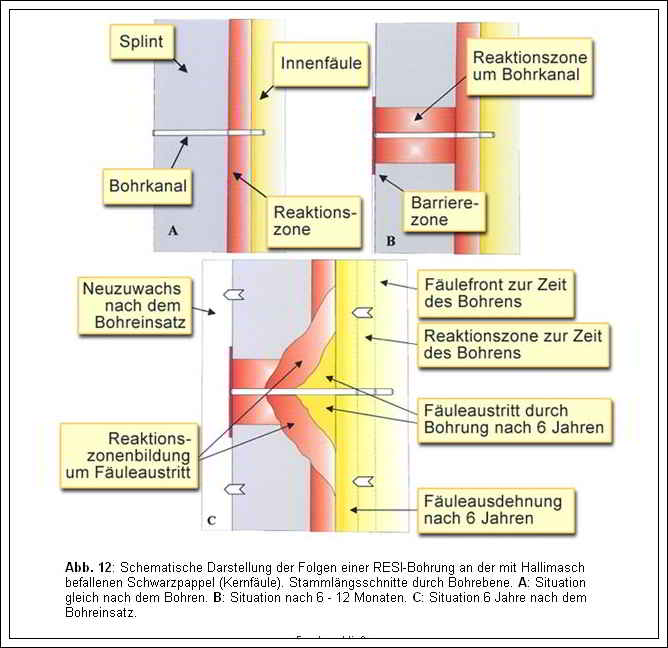
Fig. 12 Schematic representation of the consequences of RESI drilling on black poplar infested with Halli’s aspen (core rot). Longitudinal stem sections through drilling plane. A: Condition immediately after drilling. B: Condition after 6-12 months. C: Condition 6 years after drilling.
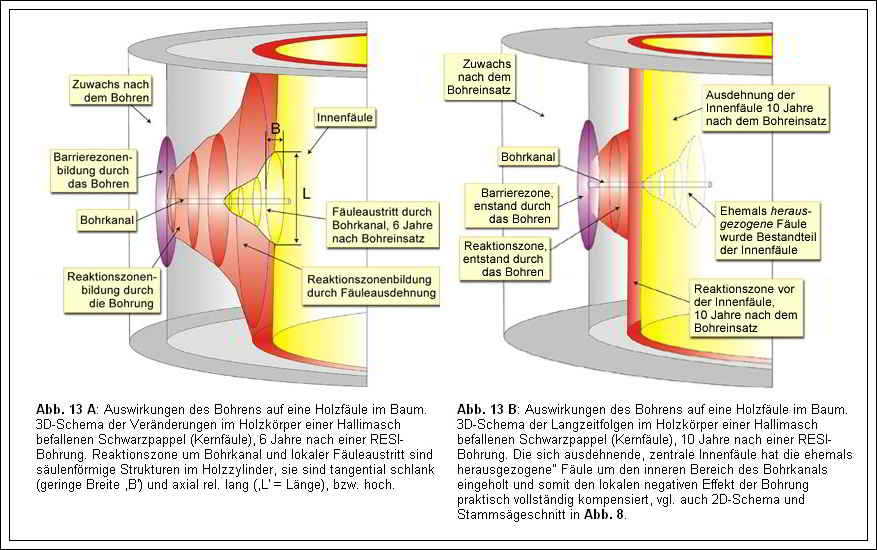
Fig. 13 a and b A: Effects of drilling on a wood rot in the tree. 3D schematic of changes in the wood body of a Hallimasch-infested black poplar (heart rot), 6 years after RESI drilling. Reaction zone around drilling channel and local rot exit are columnar structures in the wood cylider, they are tangentially slender (small width ‘B’) and axially rel. long (‘L’=length) and, respectively, high. B: Effects of drilling on a wood rot in the tree. 3D schematic of the long-term effects in the wood body of a Hallimasch-infested black poplar (heart rot), 10 years after RESI drilling. The expanding central internal rot has caught up with the formerly extracted rot around the inner area of the drilling channel, thus practically completely compensating for the local negative effect of the drilling, cf. also 2D schematic and trunk saw cut in Fig. 8.
The fact that the reaction zone formation due to decay entry in the inner drill channel area was more extensive than in the outer sapwood area was probably also due to the fact that the young outer tissue regions are more metabolically active than the inner tissue regions, which, in addition to older cells with lower activity, likely had fewer living cells (particularly parenchyma). This slower establishment of defense zones inside the tree increased the expansion of the zones internally.
At this point, we want to formulate a fundamental consideration regarding how the formation of reaction zones and barrier zones can be influenced. The more vital a tree is, the faster its living cell component in the wood responds to an injury, meaning that the containment walls (reaction zone, barrier zone) are built up more quickly, resulting in shorter and slimmer columnar discolorations in the inner stem. Vitality, however, is mainly dependent on the site conditions and the genetic factors of a particular tree species. Therefore, through the selection of resistant young trees of a specific species and optimal site selection and conditions (including maintenance), we can ensure the best possible tree-specific reaction processes.
Figure 14 shows the examined Norway maple, which exhibited extensive white rot caused by the crust fungus in the inner stem. The numerous increment and RESI drillings each triggered the formation of a barrier zone in the newly formed stemwood and a strong reaction zone in the sapwood around the drill channels. Along the increment drill channels, the internal decay began to spread from the inside out, but it was inhibited by the reaction zone surrounding the drill channel, so that the “extracted” decay only affected the inner part of the residual wall adjacent to the drill channel. These additional local decays spread caused by the many drillings had only a minor reducing effect on the resulting residual wall thickness of the tree, as shown in Figure 14, lower right image. The overall impact of drilling consequences on the stability of the stem was of secondary importance compared to the existing “natural” stem injuries.
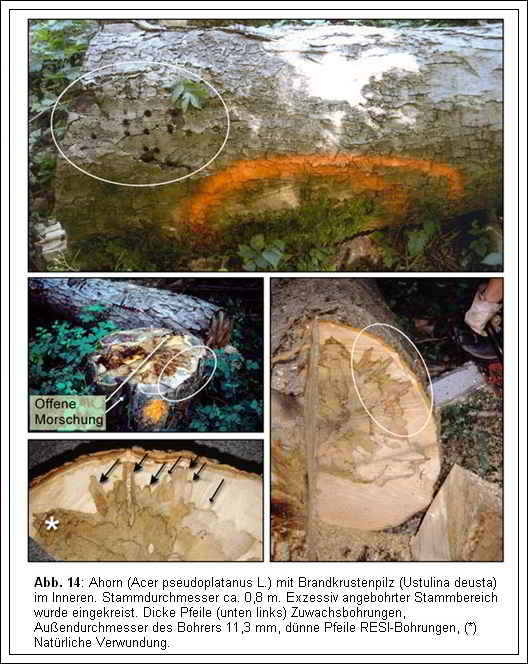
Fig. 14 Maple (Acer pseudoplatanus L.) with fire-crusted fungus (Ustulina deusta) inside. Trunk diameter approx. 0.8 m. Excessively bored trunk area was circled. Thick arrows (lower left) increment holes, outer diameter of drill 11.3mm, thin arrows RESI holes, (*) Natural wounding.
If we examine the changes in the wood tissue around each increment drilling in detail, they are qualitatively similar to those of the aforementioned RESI drillings in the heart-rotted poplar. Figure 15 displays a detailed labeled section of the stem.
Note: The translation provided assumes that “Zuwachsbohrungen” refer to increment drillings, which are typically performed to measure annual tree growth. Please verify the context to ensure the accuracy of the translation.
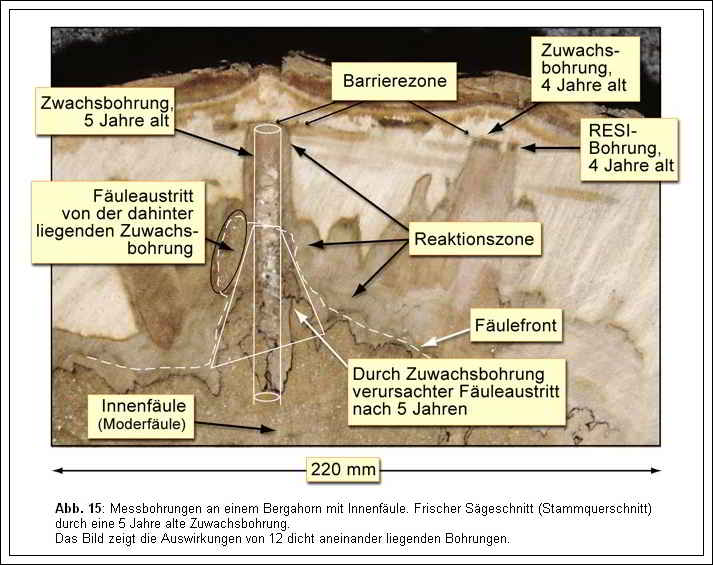
Fig. 15 Measuring holes on a mountain ahome with internal rot. Fresh saw cut (log cross section) through a 5-year-old increment bore. The picture shows the effects of 12 closely spaced boreholes.
The reaction zones of the individual drillings fused and merged together, forming a broad, continuous “decay defense wall.” When comparing Figure 15 with the cross-sectional area 10 cm away in Figure 16, the slimming of the reaction zones, meaning the tapering of the reaction zone columns around the drillings, can be observed (see 3D diagram in Figure 13 for reference). The triangular area of the “extracted” decay has also significantly reduced in size, becoming slimmer (also refer to the 3D diagram). Furthermore, in Figure 15, a direct comparison of the reaction zone formation caused by the increment drilling and the RESI drilling is possible. Both drillings were 4 years old and positioned side by side. The maple tree exhibited a qualitatively similar response to both drillings. However, quantitatively, the increment drilling (with a larger drill bit diameter) caused more significant changes, resulting in a wider barrier and reaction zone. The height of both reaction zone columns (comparison between Figure 15 and Figure 16) was approximately the same. Figure 17 illustrates the merging of multiple reaction zones in a radial longitudinal section.
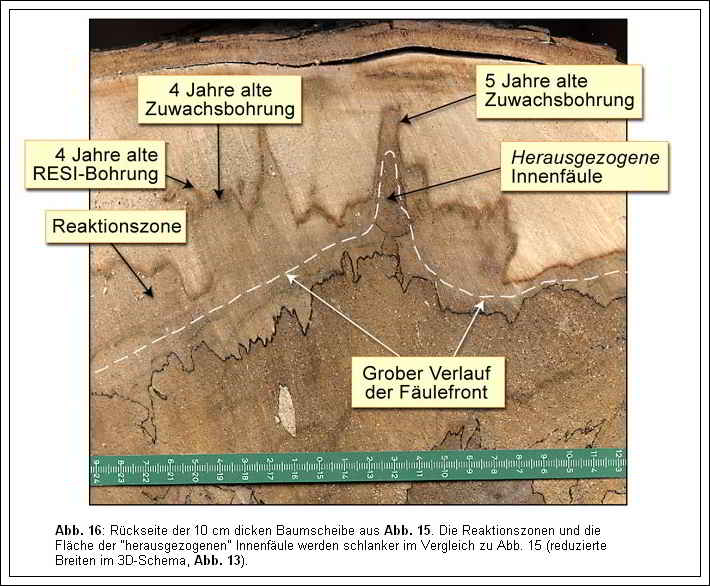
Fig. 16 Back side of the 10 cm thick tree slice from FIG. 15 The reaction zones and the area of the “extracted internal rot become slimmer compared to FIG. 15 (reduced widths in the 3D schematic, FIG. 13).
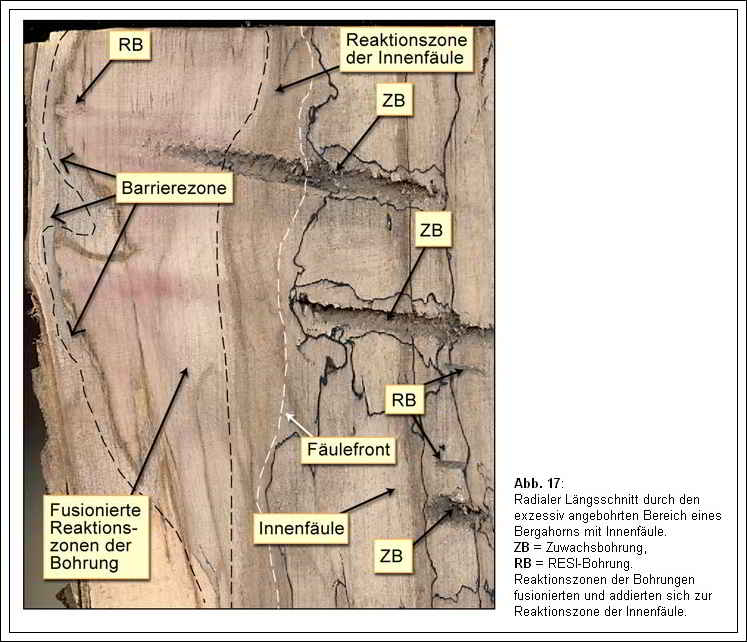
Fig. 17 Radial longitudinal section through the excessively bored area of a sycamore with internal rot. ZB = increment bore RB = RESI bore. Reaction zones of the boreholes merged and added to the reaction zone of the internal rot.
Figure 18 depicts the examined oak tree (Quercus robur L.) with a crack, exhibiting two defect symptoms: rib formation before the crack and a smaller internal decay with a swelling on the trunk preceding the decayed area. The damaged areas of the trunk have been repeatedly examined or drilled multiple times in recent years, using numerous RESI drillings and some increment drillings.
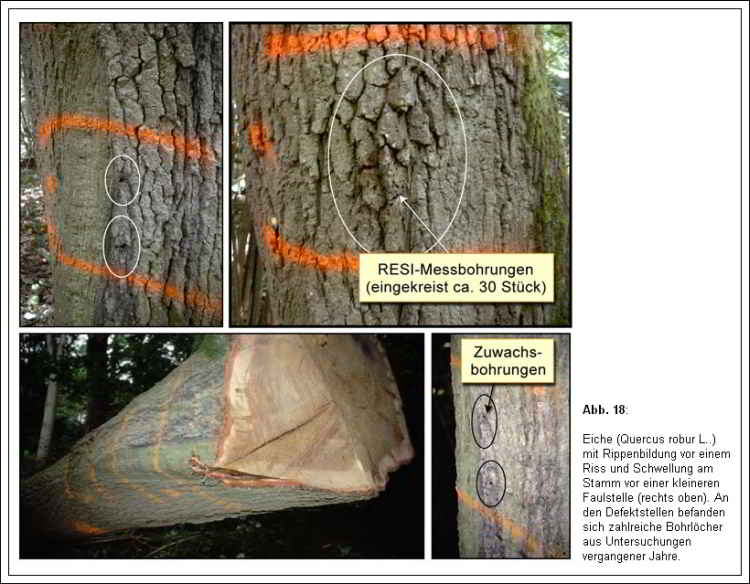
Fig. 18 Oak (Quercus robur L.) showing ribbing in front of a crack and swelling on the trunk in front of a minor rot (upper right). Numerous boreholes from investigations in previous years were located at the defect sites.
Cross-sections of the trunk through the rib revealed the presence of an internal crack, with its edges exhibiting a dark brown discoloration due to the formation of reaction zones (see Figure 19). This indicates that the crack continued to propagate in the sapwood, causing the injured living sapwood tissue to produce phenolic substances that were deposited in the cells near the crack, ultimately resulting in the brown discoloration. No wood decay was present within the crack. RESI drillings performed through the rib and the crack did not lead to any decay entry and thus had practically no consequences for the safety of the oak trunk, as shown in Figure 19.
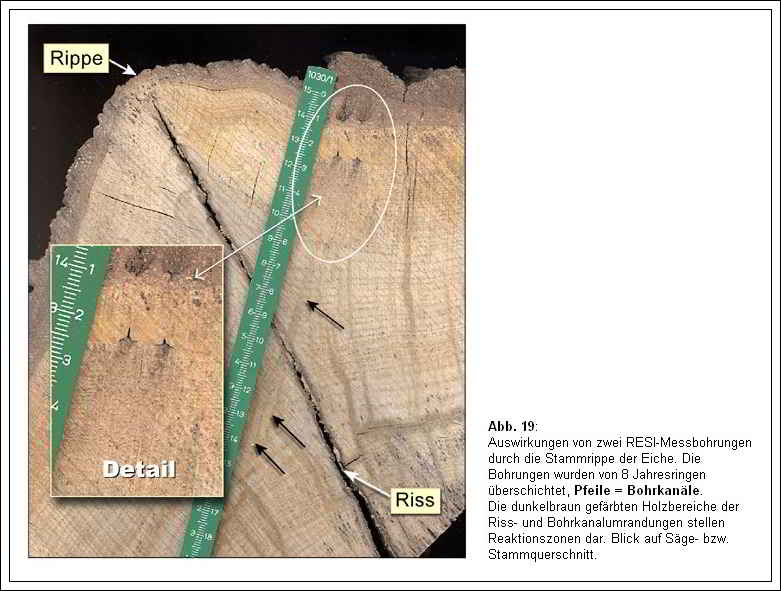
Fig. 19 Effects of two RESI measurement holes through the trunk rib of the oak tree. The drill holes were overlaid by 8 annual rings, arrows = drill channels. The dark brown colored wood areas of the crack and drill channel outlines represent reaction zones. View of saw or log cross-section.
The drilling holes were overgrown by the subsequent annual ring, and the sapwood reacted locally with the formation of reaction zones, resulting in a brown discoloration due to increased deposition of phenolic substances. Interestingly, when the saw cut through the RESI drilling was moistened at another location, as shown in Figure 20, it was observed that there was also an intense brown discoloration around the drill hole in the young heartwood. Microscopic examination clearly identified this, although not as pronounced as in the sapwood, as a reaction zone (with localized increased deposition of phenolic substances). This could only mean that there were still an adequate number of living and physiologically active parenchyma cells in the young heartwood capable of carrying out this metabolic process (similar findings have also been described in [9]). Therefore, at least for young oak heartwood, we can assume that it is still “alive” and capable of responding to injury with the formation of reaction zones, i.e., potential decay compartmentalization. It has been mentioned in the literature that even though heartwood is “dead” (or appears so), it can still respond to injuries with different discolorations: for example, Butin [6] described discoloration of heartwood after injury as “oxidation discoloration,” where the penetrating oxygen reacts with the phenolic cell constituents produced by so-called heartwood formation, resulting in a gray-brown “stain.” Holdenrieder [7] mentioned that chemical reactions also occur in dead heartwood, inhibiting the spread of fungi. Shigo [4] stated that heartwood can also respond to injury by forming boundary zones capable of encapsulating pathogens. Pearce [9] even wrote that phenol-oxidizing enzymes can remain active in the cell walls of dead heartwood cells.
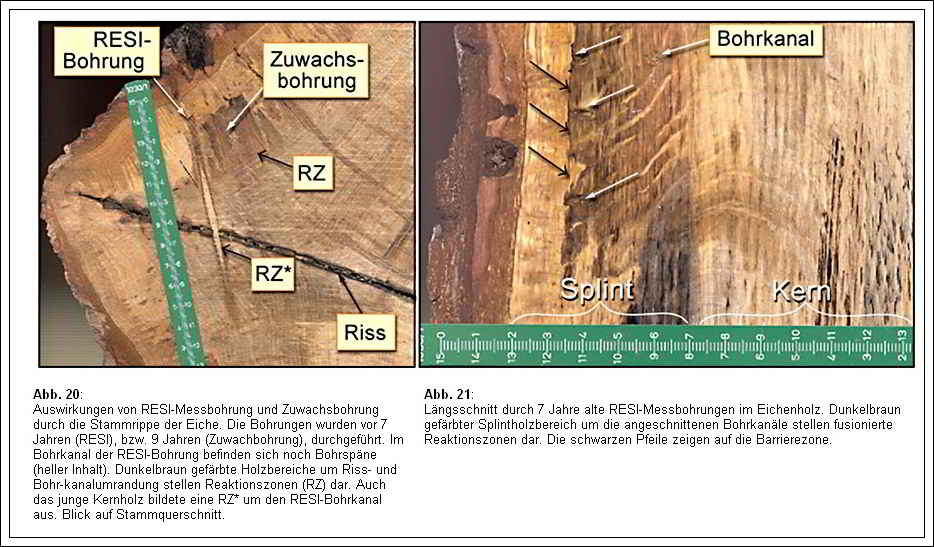
Fig. 20 Effects of RESI measurement drilling and increment drilling through the trunk rib of the oak. The boreholes were drilled 7 years ago (RESI) and 9 years ago (increment borehole). Drilling chips (light-colored content) are still present in the drilling channel of the RESI borehole. Dark brown colored wood areas around crack and drill channel perimeter represent reaction zones (RZ). The young heartwood also formed a RZ around the RESI drill channel. View of log cross section Fig. 21: Longitudinal section through 7-year-old RESI monitoring wells in oak wood. Dark brown colored sapwood area around the incised drill channel represents fused reaction zones. The black arrows point to the barrier zone.
The reaction of the oak tree to the growth drilling was qualitatively equivalent to the reaction to the RESI drilling. Figures 20, 23, and 24 show longitudinal and cross-sections through growth drilling channels. Since the diameter of the growth drill bit was larger than that of the RESI needle, the drilling hole and thus the extent of the injury or the discolored zones were larger. We observed an intense formation of reaction zones around the drilling hole in the sapwood and only a weak formation in the young heartwood. No decay penetrated the wood through any of the growth drillings.
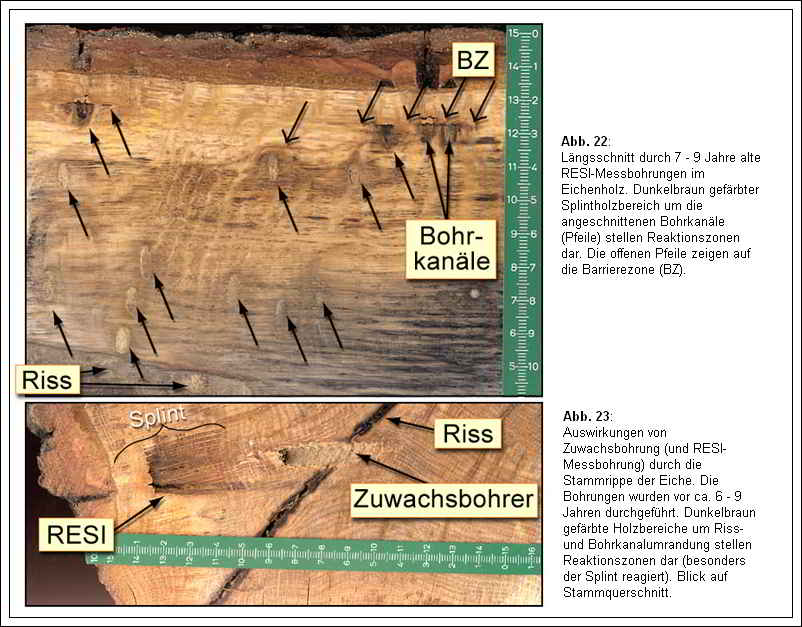
Fig. 22 Longitudinal section through 7–9-year-old RESI monitoring wells in oak. Dark brown colored sapwood area around the cut drilling channels (arrows) represent reaction zones. The open arrows point to the barrier zone 23 Effects of increment drilling (and RESI measurement drilling) through the stem rib of oak. The drilling was carried out about 6-9 years ago. Dark brown colored wood areas around crack and drill channel perimeter represent reaction zones (especially sapwood reacts). View of trunk cross-section.
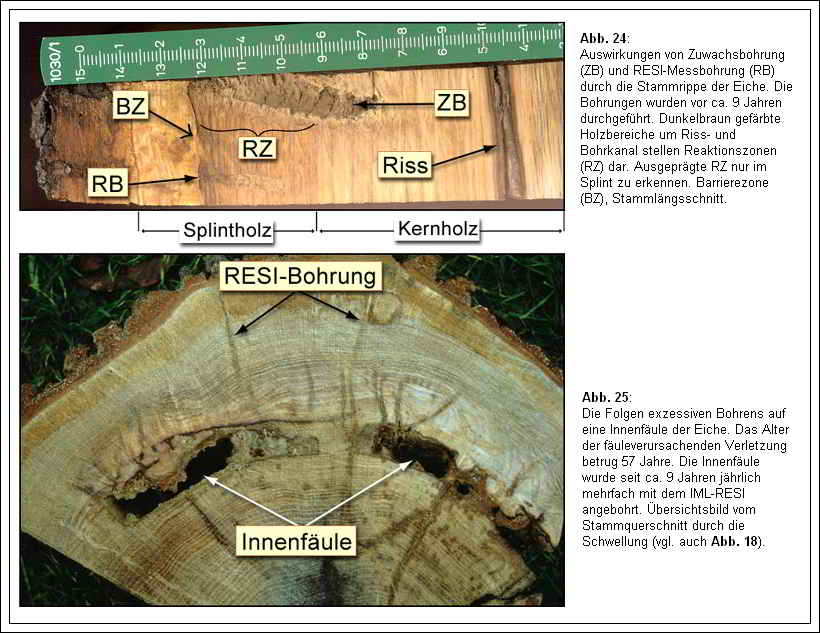
Fig. 24 Effects of increment drilling (ZB) and RESI measurement drilling (RB) through the stem rib of oak. The boreholes were drilled about 9 years ago. Dark brown colored wood areas around crack and drill channel represent reaction zones (RZ). Pronounced RZ only visible in sapwood. Barrier zone (BZ), log longitudinal section 25 The consequences of excessive drilling on an internal rot of the oak. The age of the rot-causing injury was 57 years. The internal rot was drilled several times a year with the IML-RESI for about 9 years. Overview image of the trunk cross-section through the swelling (see also FIG. 18).
Figures 21, 22, and 24 show longitudinal sections with barrier zone formation. The consequences of excessive drilling on the internal decay of the oak tree are depicted in Figures 25 and 26. The tree effectively compartmentalized the internal decay (see Fig. 26: Walls II, III, IV) and the strong drilling intervention (IML-RESI) over the past 9 years did not spread it outward into the healthy wood. The age of the decay-causing injury was 57 years. Furthermore, the RESI drilling in the stem swelling (or internal decay) did not cause any external decay entry in any case, ultimately having practically no negative consequences for the safety of the oak tree.
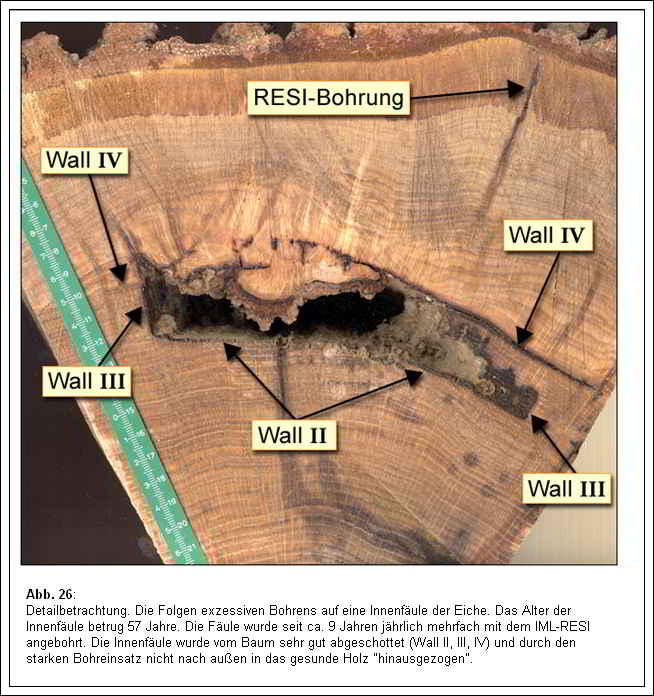
Fig. 26 Detail Consideration. The consequences of excessive drilling on an internal rot of the oak. The age of the internal rot was 57 years. The rot was drilled several times a year with the IML-RESI for about 9 years. The internal rot was very well compartmentalized by the tree (Wall II, III, IV) and was not “pulled out” into the healthy wood due to the heavy drilling.
Finally, Figure 27 shows a leaning sycamore tree (Acer pseudoplatanus L.) with numerous growth drilling on both the tension and compression sides of the bend at the base of the trunk. It did not have any internal decay before drilling. The growth drilling was conducted 9 years prior to felling and involved complete through-drilling of the trunk. Additionally, the trunk had several RESI measurement drillings.
If a decay-causing pathogen had penetrated the healthy trunk through excessive drilling and managed to establish itself within 9 years, there should be internal wood decay or even a cavity inside. Pure wood discoloration caused by drilling does not represent wood decay or fungal rot and can now (after 9 years) be clearly distinguished from genuine wood decay, which, if present, would have reached an advanced stage by now.
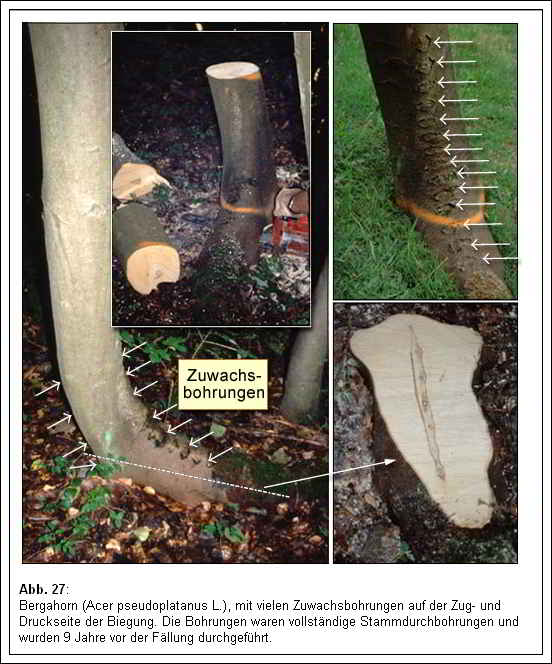
Fig. 27 Sycamore maple (Acer pseudoplatanus L), with many increment holes on the tension and compression side of the bend. The drillings were complete stem drillings and were carried out 9 years before felling.
Figures 28-32 depict several cross-sectional and longitudinal cuts of the excessively drilled sycamore tree from different planes and perspectives. The trunk, which was free of decay before drilling, remained decay-free even nine years after drilling. No wood decay penetrated the trunk’s wood. As shown in Figure 28, blue stain fungi had entered through a growth drilling vertically into the horizontal basal part of the trunk and established themselves in the dead wood area around the hole. These so-called blue stain fungi cause a blue-black discoloration of the wood. They are inhabitants of dead wood but not wood destroyers. According to Schmidt[8], blue stain fungi practically do not attack the cell wall and have little impact on wood strength because they feed only on cell contents. The wood discolors because the thick hyphae of the fungi are brown due to melanin and shine through the wood tissue.
The presence of blue stain fungi at a drilling hole indicates that ubiquitous fungal spores can indeed enter the drill holes and germinate, but it is rare for the fungi to establish themselves there. It can be assumed that fungal spores (including those of wood-decaying fungi) also entered the other drilling holes, but they were unable to establish themselves there.
All these observations consequently lead to the conclusion that growth and RESI drilling pose generally no significant risk of wood decay infection for healthy trees.
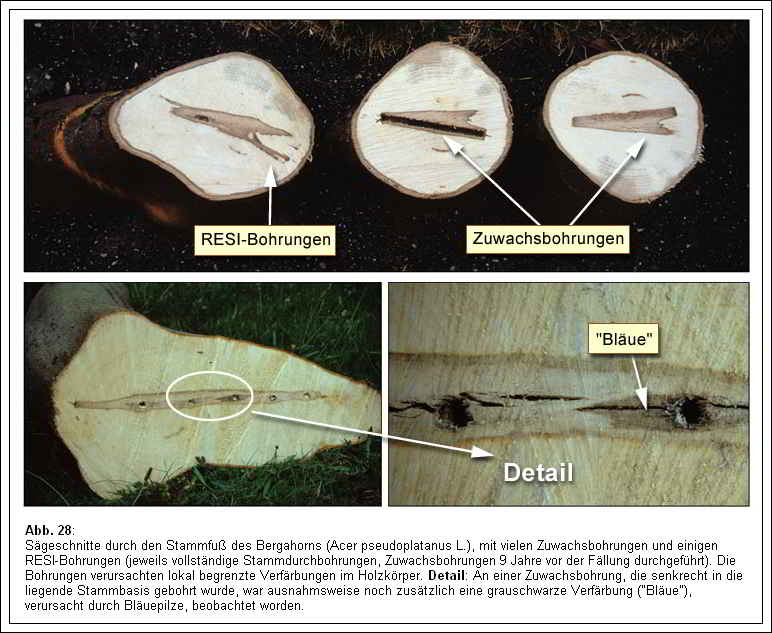
Fig. 28 Saw-cuttings through the stem base of sycamore (Acer pseudoplatanus L.), with many increment drills and some RESI drills (complete stem drills in each case, increment drills made 9 years prior to felling). The borings caused localized discoloration in the woody body. Details: At one increment hole, which was drilled vertically into the lying trunk base, a gray-black discoloration (“blue stain”), caused by blue stain fungi, was exceptionally observed.
Figure 29 A shows the tree’s reaction to the drillings on a trunk cross-section. The wounded superficial tissue layer around the drill hole died off, and immediately behind it, a reaction zone formed, which closely followed the shape of the drill hole in the cross-section. In the longitudinal section, Figure 29 B, the axial extent of the reaction zones can be observed, which is significantly larger than the tangential extent, as well as the fusion of the zones in closely spaced drillings. Figures 29 C and 29 D depict the closure of the drill holes through callus tissue formation. The effects of both drilling methods can be directly compared in Figure 30. The RESI drillings caused significantly less discoloration (formation of reaction zones) compared to the growth drillings. Both methods also triggered the formation of barrier zones, as shown in Figures 31 and 32 (also refer to Figure 29 B).
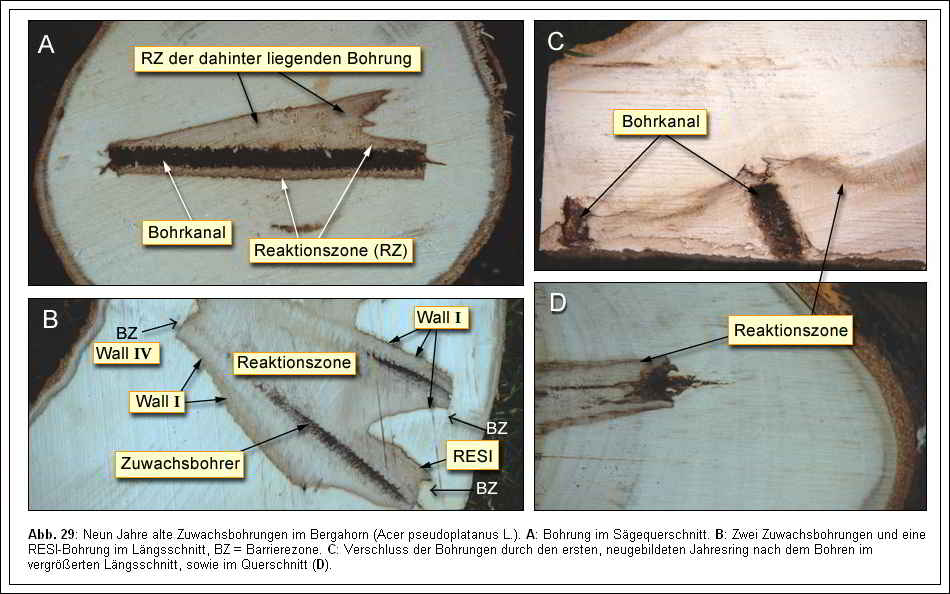
Fig. 29 Nine-year-old increment borings in sycamore (Acer pseudoplatanus L). A: Bore in saw cross section. B: Two increment bores and one RESI bore in longitudinal section, BZ = barrier zone. C: Closure of the holes by the first, newly formed growth ring after drilling in enlarged longitudinal section, as well as in cross section (D).
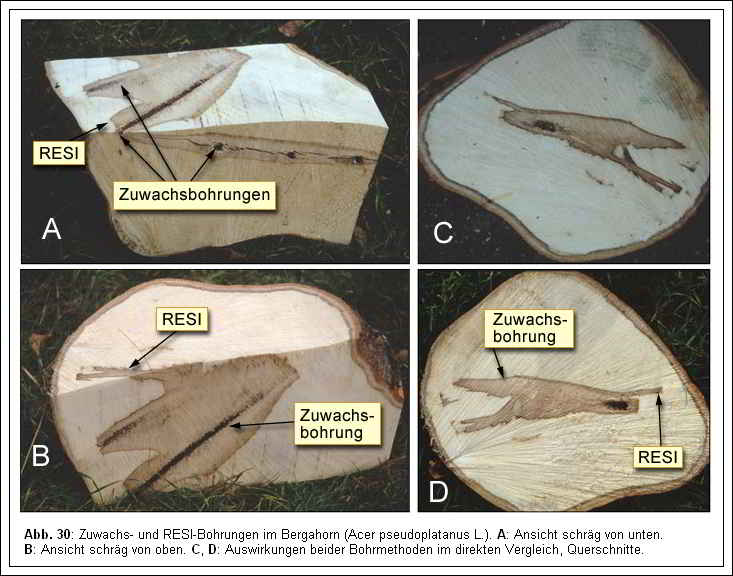
Fig. 30 Increment and RESI drilling in sycamore (Acer pseudoplatanus L.). A: Angled view from below. B: View diagonally from above. C, D: Effects of both drilling methods in direct comparison, cross sections.
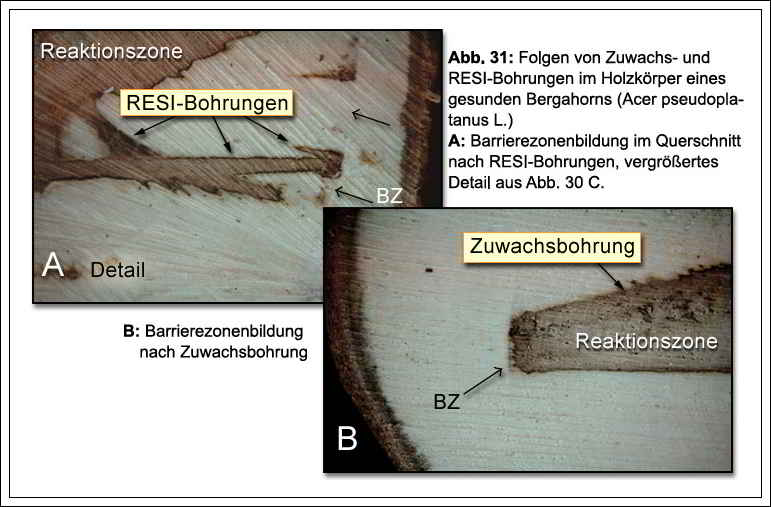
Fig. 31 Consequences of increment and RESI drilling in the wood body of a healthy sycamore maple (Acer pseudoplatanus L.). A: Barrier zone formation in cross section after RESI drilling, enlarged detail from Fig. 30 C. B: Barrier zone formation after incremental drilling.
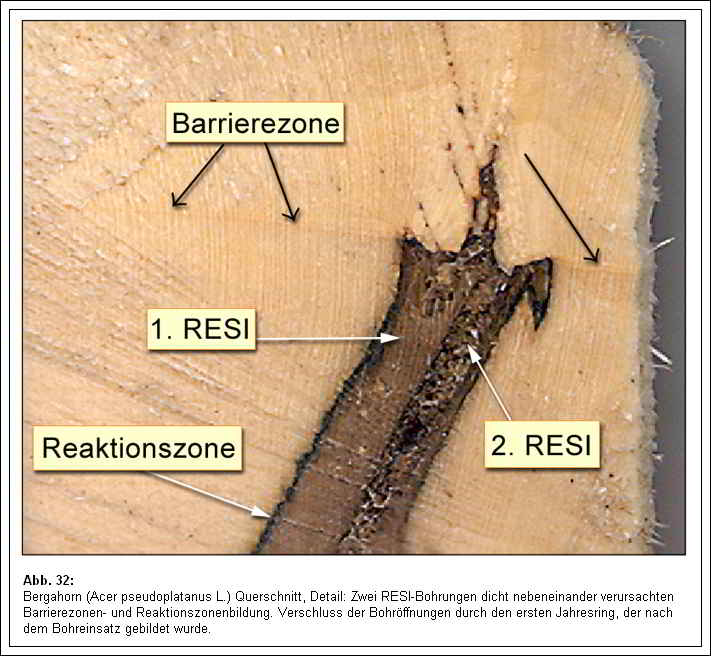
Fig. 32 Sycamore (Acer pseudoplatanus L.) cross-section, details: two RESI wells close together caused barrier zone and reaction zone formation. Closure of the drill holes by the first growth ring formed after drilling.
The wood areas described here as reaction zones in the maple tree trunk consisted of two differently colored zones. A dark brown zone, which exhibited pronounced phenolic deposits and plugs for vessel occlusion, delimited the entire discolored zone towards the outer lighter wood tissue. Between the drilling channel and the dark brown zone, there was a light brown zone that had fewer phenolic deposits and minimal plugs. The phenolic deposits were predominantly found in the wood ray parenchyma cells within this light brown zone. This was likely relatively rapidly deceased wood tissue following the drilling injury (at the beginning of the nine-year experimental period), which produced and deposited phenolic antimicrobial substances, mainly in the longer-living wood ray cells. The presence of these phenolic substances defined this zone as a reaction zone, as these substances could not be detected in the adjacent light wood, including the core region. However, this zone does not represent a typical core-forming process since Acer pseudoplatanus does not form heartwood. One could refer to this zone as an old, inner, embolism-damaged reaction zone, in contrast to the fresh, dark brown-colored, outer reaction zone.
With the previous explanations, we have been able to answer the initial three questions, namely how a tree with internal decay and a tree without internal decay react to excessive drilling. An important fact that has become clear from the investigations is that we should never equate discoloration with decay. Discoloration does not necessarily indicate decay, and we should never equate decay-causing agents and decay, i.e., cause and effect. An existing decay-causing agent in the drilling channel (fungal spore or hyphae), for example, does not necessarily have to establish itself there and cause wood decay, as the tree can successfully isolate or defend against the decay-causing agent.
Now it is necessary to evaluate the consequences of drilling in terms of their danger. The experimental trees were vital both before and 8-10 years after the initial drilling. Drilling did not result in any noticeable loss of vitality for the trees. The reduction in stability and resistance to breakage in the experimental trees with internal decay was primarily determined by the internal decay itself, so the locally and temporarily formed “funnel-shaped” decay cones resulting from the drilling did not significantly contribute to the overall reduction in stability and resistance to breakage. These findings suggest that the drilling techniques used, which are also only applied in a limited and non-excessive manner in practice, are not dangerous to the tree overall. Since the increment borer had a larger diameter than the RESI drilling needle, it caused larger discolorations in the experimental trees, but this did not make it significantly more or less dangerous overall.
Does the tree inspector need to drill? If they encounter a warning signal on the tree, such as fruiting bodies of wood-decaying fungi or swelling on the trunk, they must conduct a thorough investigation. According to legal practice, they would be considered negligent if they fail to do so. For example, if a tree inspector has a reasonable suspicion of internal decay (e.g., due to bulges on the trunk), they must determine the remaining wall thickness to apply criteria for assessing the potential danger. Currently, the most reliable determination of remaining wall thickness and wood quality is only possible through the sensible application of drilling techniques. Therefore, when there is a reasonable suspicion, the tree inspector must drill to verify the danger posed by the tree or, alternatively, preemptively eliminate any potential danger to people by felling the suspicious tree. If the only alternatives to drilling are felling, any tree-friendly inspector would choose to use drilling techniques.
Dr. K. Weber and Prof. Dr. C. Mattheck
Forschungszentrum Karlsruhe GmbH
in der Helmholtz-Gemeinschaft
Institut für Materialforschung II
Postfach 3640
D- 76021 Karlsruhe
The original article in German was written by Dr. K. Weber and Prof. Dr. C. Mattheck, and the translation is provided by IML. Information is provided without guarantee.